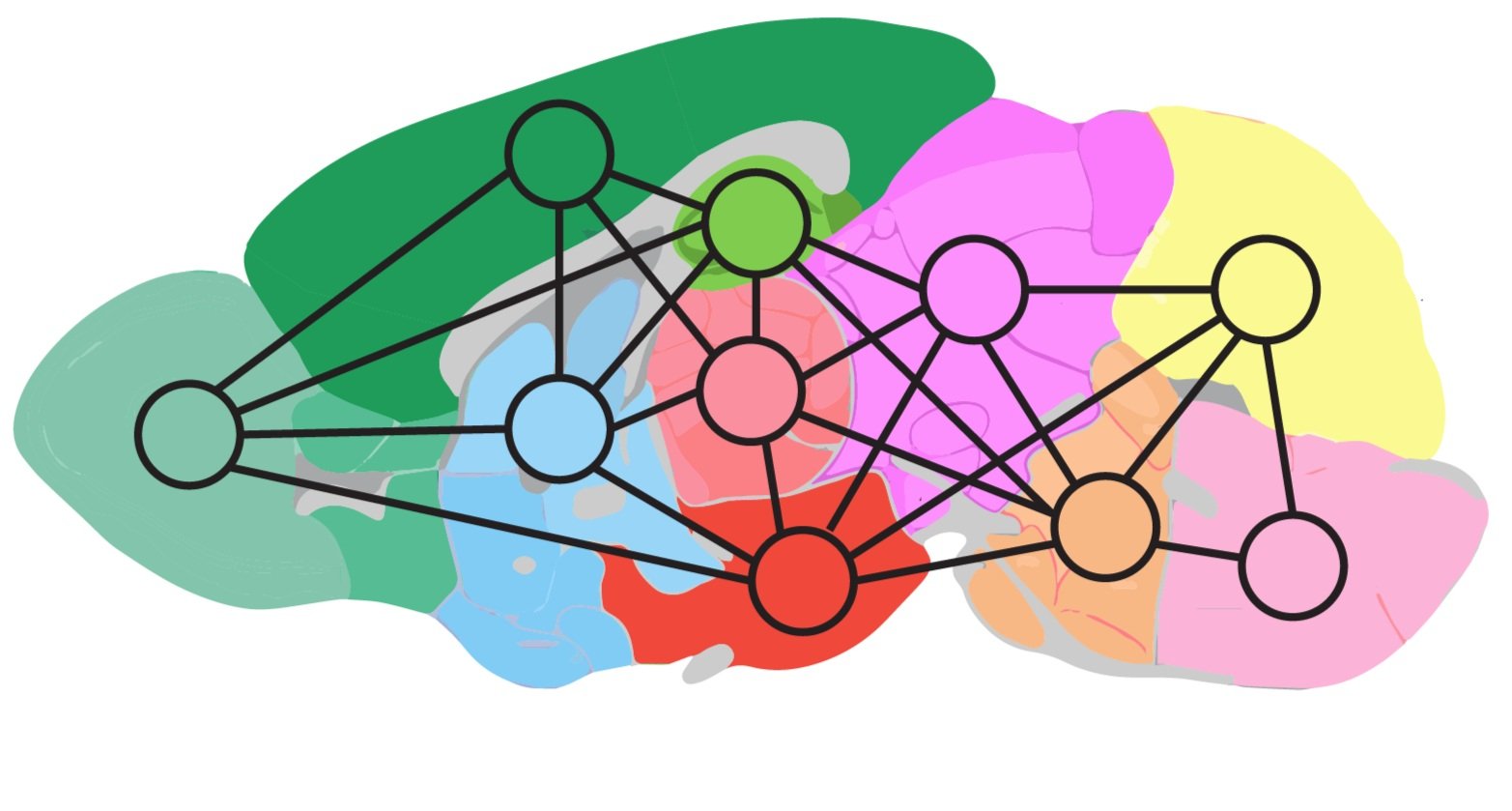
Research
The Gomez lab uses molecular biology, electrophysiology, and functional imaging to decode the instructive cues that organize neural networks. We focus on mechanisms that specify synaptic properties and determine how these properties bias the timescales of neuronal computation. We are working to discover how synaptic dysfunction manifests in conditions like autism, intellectual disability, and neurodegeneration.
Alternative RNA splicing and neural function:
We share the same number of genes as our vertebrate relatives, demonstrating that gene number does not scale with brain complexity. Therefore, we look to alternative RNA splicing for its potential to vastly expand proteomic diversity. Given that 95% of multi-exon genes are alternatively spliced and the extent of alternative splicing scales with network complexity, we focus on how alternative splicing shapes cell type transcriptomes for neuronal function. How does this potential increase in molecular complexity operate selectively in neural networks? To answer this, we query the output of splicing regulators that act in nonoverlapping cell populations in the brain using two strategies: 1) we identify cell type-specific transcriptional fingerprints tuned by alternative splicing; and 2) we link these RNA encoded cues to cell type-specific dynamics of ionic flux.
The RNA biology of psychedelics
In neurological disorders, our ability to move, learn, remember, empathize, or recover from trauma is not limited by will but by the capacity of our neurons to synthesize components for cognitive flexibility. Plasticity in the brain counters this rigidity, yet we lack insight into opening plasticity windows in the brain. Psychedelics have been used in Indigenous healing practices for millennia to engage cognitive connection and open pathways to healing. We synthesize two rapidly evolving fields - RNA biology and psychedelics - to discover novel plasticity RNAs and understand how they coordinate large-scale neural plasticity.
Uncovering the cellular basis for input-specific responses and their role in neuronal integration:
CA1 pyramidal neurons in the hippocampus receive tens of thousands of inputs from different brain regions, each with unique synaptic properties. These inputs are organized and processed in discrete subcellular compartments, generating distinct dynamics of ionic flux necessary for synaptic integration and neuronal computation. However, it is unclear how input-specific cellular responses coordinate changes to synapses from discrete inputs and alter local and global time windows for integration. To better understand these responses, we combine functional imaging and electrophysiology to observe the local and global rules that emerge from transsynaptic signaling.
Can an axon multiplex information that depends on the identity of its target?
A single axon from a CA3 pyramidal neuron in the hippocampus can terminate onto 30,000 targets that include different cell types. Little is known about how presynaptic components distribute to a neuron’s many axon terminals, despite knowing that synaptic properties can differ depending on the target neuron. Considering the various targets of CA3 pyramidal neurons, how are distinct synaptic properties established? We use cell type-specific genetic strategies to reveal the cellular rules by which single neurons communicate with different targets.
Donate
Join us in advancing our understanding of neural function. Click the button below to contribute to our ongoing efforts.